August 17, 2017 saw a major breakthrough in astronomy, when gravitational waves from a pair of colliding neutron stars were detected for the first time by the US-based Laser Interferometer Gravitational-Wave Observatory (LIGO) and the Europe-based Virgo detector. The detection was confirmed by a large number of telescopes around the world that studied various forms of radiation from the merger, marking the beginning of gravitational wave multi-messenger astronomy.
Press Release
First detection of gravitational waves from colliding neutron stars
This movie shows the LIGO and VIRGO observations of the gravitational wave GW170817. In the LIGO Hanford and LIGO Livingston panels, GW170817 appears as a blue-green/yellow curve. This "chirp" signal turns sharply upward near time 0 seconds, which is 5:41:04AM PDT on August 17, 2017. The movie converts a combination of the observed stretching and squeezing of the LIGO Hanford and LIGO Livingston detectors into sound at the same frequencies.
Credit: LIGO/Virgo/Lovelace, Brown, Macleod, McIver, Nitz.
Comparison of GW170817 signal to binary black hole merger signal detected before it. All waveforms are the inferred signals incident on the Hanford detector, and are shown from 30 Hz to merger. Signal from the neutron star merger was expectedly much longer than the black hole merger signals, though the total energy emitted by black hole mergers is much more compared to neutron star mergers.
The masses of stellar remnants are measured in many different ways. This graphic shows the masses for black holes detected through electromagnetic observations (purple), the black holes measured by gravitational wave observations (blue), neutron stars measured with electromagnetic observations (yellow), and the masses of GW170817 measured by gravitational wave observations (orange). The remnant of GW170817 is unclassified, and labeled as a question mark. Credit: LIGO/Virgo/Northwestern/Frank Elavsky
The beginning of gravitational wave multi-messenger astronomy
United effort by Astronomers Worldwide
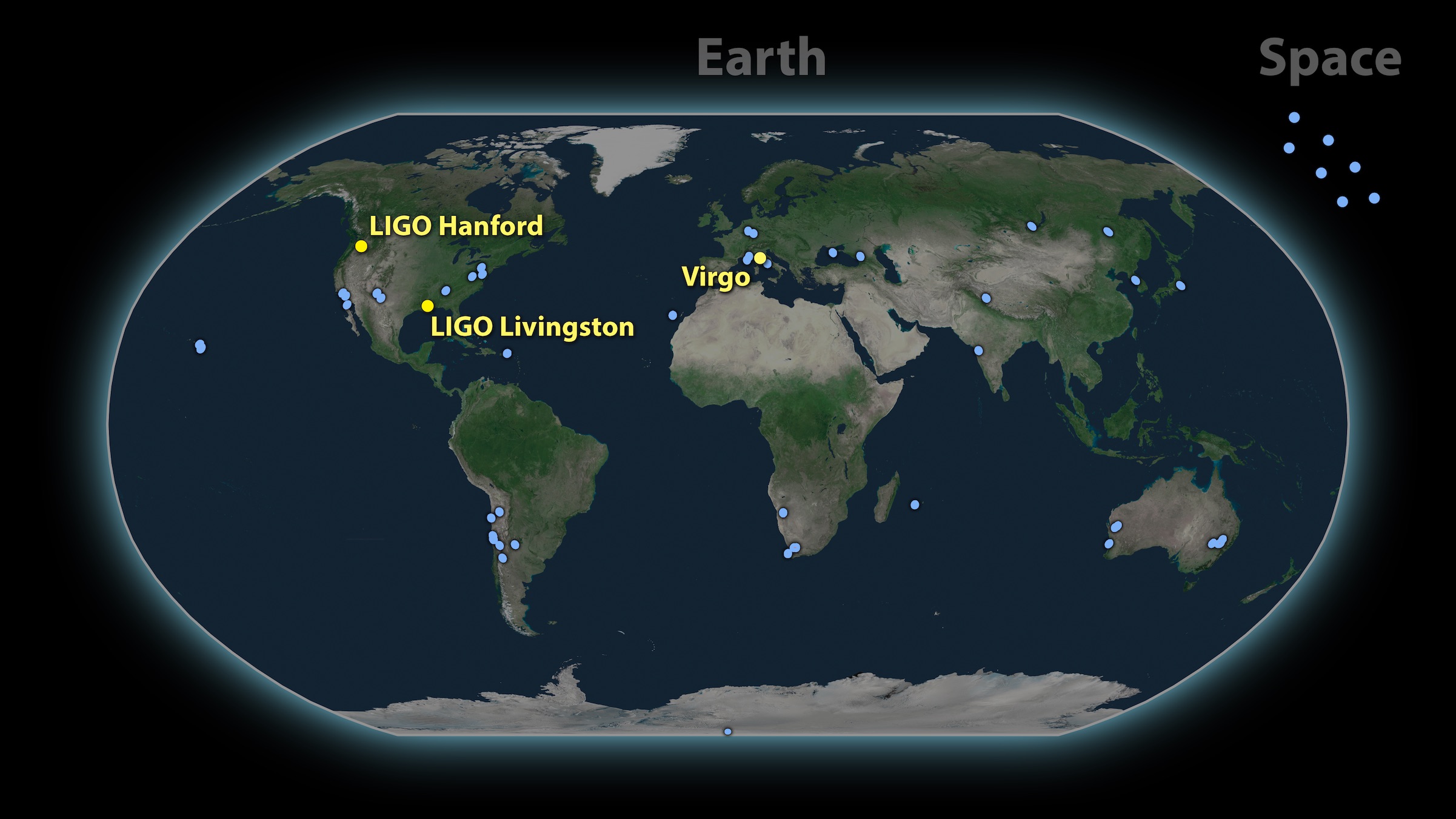
Followed up the event for several days
Together they could pinpoint the host galaxy
Studied the source in multi-wavelength
Confirmed that GRBs are caused by BNS mergers
The short clip shows the merger of two neutron stars with 1.34 and 1.25 solar masses. The blue material is the matter which becomes unbound and leaves the system. The most likely scenario is that ejected matter from neutron star mergers is the main source of heavy elements in the universe. Credit: T. Dietrich (Friedrich Schiller University Jena and Max Planck Institute for Gravitational Physics); BAM collaboration. The BAM collaboration is an international collaboration between the Florida Atlantic University, Friedrich Schiller University Jena, Istituto Nazionale di Fisica Nucleare, Max Planck Institute for Gravitational Physics, Università di Parma, Universidade Federal do ABC.
The relative probability of different values of Hubble Constant (H0), the local expansion rate of the universe, is represented by the solid blue curve, which peaks at 70 km/s/Mpc. The dashed and dotted blue vertical lines respectively show the limits of 68.3% and 95.4% credible intervals for H0. The vertical green and orange bands represent the constraints on H0 from two state-of-the-art analyses using solely electromagnetic data: the green bands show the range of values inferred from analysis of CMBR data obtained by the Planck satellite; the orange bands show the range of values inferred from the SHoES analysis that combines Cepheid variable and type Ia supernovae data from the relatively nearby universe. The darker and lighter coloured bands indicate 68.3% and 95.4% credible intervals respectively. Note that the Planck and SHoES results are not in agreement with each other at the 95.4% probability level. Our gravitational-wave result is, however, consistent with both the Planck and SHoES values.
Animation revealing the LIGO and Fermi-GBM data synchronised so to the merger time of the neutron stars. The GRB occurred two seconds after the merger. The audio is the "chirp" sound of the gravitational wave signal, followed by a "ding" at the GRB time. Credit: NASA GSFC & Caltech/MIT/LIGO Lab
The small time delay between the arrival of gravitational waves and gamma rays tells us that gravitational waves travel nearly at the speed of light, the fractional difference is less than one part in 1,000,000,000,000,000.
LIGO + Fermi Gamma ray Burst Monitor joint detection
NGC4993 color composites (1.5' x 1.5'). Left: Composite of detection images, including the discovery z image taken on 2017 August 18 00:05:23 UT and the g and r images taken 1 day later; the optical counterpart of GW170817 is at RA,Dec = 197.450374, -23.381495. Right: The same area two weeks later. Credit: Soares-Santos et al. and DES Collaboration
Swope and Magellan telescope optical and near-infrared images of the first optical counterpart to a gravitational wave source, SSS17a, in its galaxy, NGC 4993. The left image is from August 17, 2017, 11 hours after the LIGO/Virgo detection of the gravitational wave source, and contains the first optical photons of a gravitational wave source. The right image is from 4 days later. SSS17a, which is the aftermath of a neutron star merger, is marked with a red arrow. On the first night, SSS17a was relatively bright and blue. In only a few days, it faded significantly and its color became much redder. These observations show that heavy elements like gold and platinum were created in the neutron star merger. Credit: 1M2H/UC Santa Cruz and Carnegie Observatories/Ryan Foley
LIGO-India
The planned LIGO-India detector
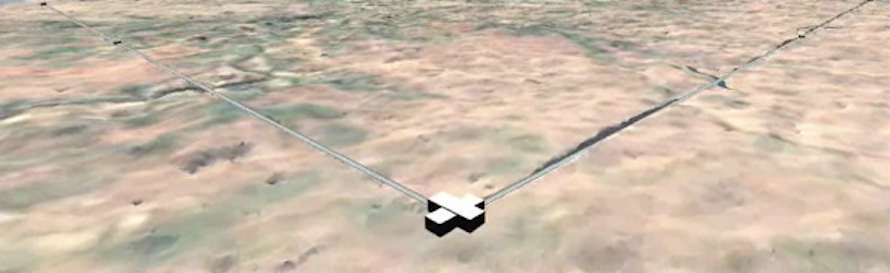
Is funded by the Department of Atomic Energy (DAE) and the Department of Science & Technology (DST), and is likely to start operating in 2024. The project is being executed by four Indian lead institutions, Inter-University for Centre for Astronomy & Astrophysics (IUCAA), Institute of Plasma Research (IPR), Raja Ramana Centre for Advanced Technology (RRCAT) and Directorate of Construction & Estate Management (DCSEM).
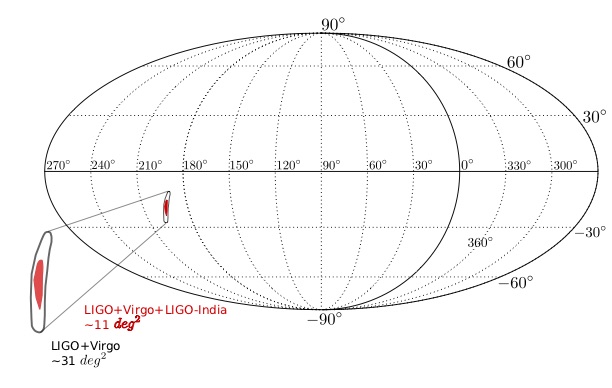
LIGO-India will introduce many-fold improvement in source localisation. Had LIGO-India been operating during GW170817, the localisation accuracy would have been about three times better than the LIGO-Virgo network. This will enable a much faster localisation of the source and follow-up right from the first moments in every frequency band of the electromagnetic spectrum. Credit: J. Rana, IUCAA
Contribution by IUCAA members
Contribution to Gravitational Wave Detection
- Matched Filtering
- A key analysis technique needed for every detection so far, pioneered at IUCAA
- Several refinements to the original analysis (e.g., to include multiple detectors)
- Development of efficient analysis techniques
- Network Analysis
- Optimal strategy to search for a signal in data from multiple detectors
- Detector Characterisation
- Application of Machine Learning to classify instrumental & astrophysical transients
- Development of an earthquake monitoring system
- Development of digital filters for LIGO seismic control system
- Application of Machine Learning for rapid documentation search
- Hosted at: http://heyligo.gw.iucaa.in
- Efficient methods for handling instrumental artefacts
- Developing search algorithms for Stochastic backgrounds
- A stochastic background from binary black-holes may be detectable by advanced LIGO in the next few years
- Operating a LIGO Tier-2 data centre at IUCAA
- Being used by national and international collaboration members
- Towards building a LIGO detector in India
- To dramatically improve the accuracy for estimation of location and orientation
11 researchers are part of the GW170817 LIGO-Virgo discovery paper. They are Anirban Ain, Sukanta Bose, Sanjeev Dhurandhar, Bhooshan Gadre, Sharad Gaonkar, Sanjit Mitra, Nikhil Mukund, Abhishek Parida, Jayanti Prasad, Tarun Souradeep, Jishnu Suresh.
Contribution to Electromagnetic Follow-up
IUCAA scientists were instrumental in searching for electromagnetic emission from the binary. They include Dipankar Bhattacharya, Javed Rana, Gulab Dewangan, Ajay Vibhute and Rupak Roy. The sensitive CZTI instrument on AstroSat was used to help narrow down the location of the gamma-ray flash. AstroSat was launched in 2015 by the Indian Space Research Organisation. Additionally, Rana contributed to the finding of radio emission with the Very Large Array radio observatory in New Mexico and Roy contributed to follow up with the extended-Public ESO Spectroscopic Survey for Transient Objects (ePESSTO) in Chile.
Publications with IUCAA members as authors
- LIGO-Virgo detection (Physical Review Letters)
- Multi-Messenger Astronomy (Astrophysical Journal Letters)
- GW-GRB connection (Astrophysical Journal Letters)
- EM follow-up including Astrosat (Science)
- EM follow-up with VLA, Caltech-NRAO & JAGWAR (Science)
- Follow-up involving ePESSTO (Nature)
- Hubble constant (Nature)
- Kilonova interpretation (Astrophysical Journal Letters)
- Progenitor of Binary Neutron Stars (Astrophysical Journal Letters)
- Implications for the Stochastic background
- Neutrino Multimessenger Observations